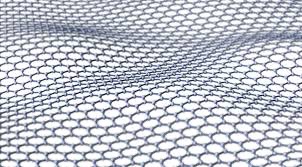
A microbial fuel cell [MFC] is a chamber divided by a membrane into anode and cathode. Bacteria grow on the anode and convert the substrates into carbon dioxide, protons and electrons. The electrons that are produced are then transferred via an external circuit to the cathode chamber, while the protons pass through the membrane. In the cathode chamber, a reaction between the protons and the electrons uses up oxygen and forms water. And as long as substrates are continually converted, electrons will flow – which is what electricity is. [1] It seems that small cells connected in series offer higher potentials than bigger reactor volumes. [2] This is not at all new, even though it may seem so (Michael Potter, 1911 [5]). Generally, the problem seems to be the lifetime of the biofuel cell. Table 5 of [4] reports on 'typical biofuel cells reported recently', where some lifetimes are measured in hours, but those labelled glucose/O₂ seemed to offer promise.
Generally, [5], a wide variety of organic material will work as fuel; tehy can be achieve efficiencies over 50% (easily better than a combustion engine); better than a hydrogen cell; they don't work well in the cold, preferring 20-40ºC; they can be made very small; they can quite easily be small, renewable and not need recharging (which sounds too good to be true). At industrial scale, there is not enough data to be helpful, where there is an obvious demand for non-toxic, cheap electricity supply, even if potentially massive. The challenge is to produce plant microbial fuel cells at scale. Do read [5].
Related in nature is a biobattery, [7] where the reaction at the anode breaks down sugar
Glucose → Gluconolactone + 2H+ + 2e− and at the cathode there is a reduction reaction to produce water,
O2 + 4H+ + 4e− → 2H2O. If there is a continuous supply of sugars, a biobattery will keep on running without any external power supply. However they are not good at retaining energy, so they tend towards uses where the demand is instant - generation rather than storage. ¹
Another bacteria of interest is a newly discovered bacterium, Shewanella oneidensis, dubbed "Electric Bacteria" which can reduce toxic manganese ions and turn them into food.[5] In the process it also generates electrical current, and this current is carried along tiny wires made of bacterial appendages called bacterial nano-wires. This network of bacteria and interconnected wires creates a vast bacterial biocircuit unlike anything previously known to science. Besides generating electricity it also has the ability to store electric charge. [7] and here. I find the terminology opaque, but I think the ability to store charge is quite short, perhaps up to an hour, which suggests to me that this would lend itself to continuous or batch supply of suitable biological material. Again, I find myself thinking of waste products, from biomass to sewage.
The clever toilet in the top picture has an example in Ghana, which generated enough power to run a light in the loo, while helping the composting of the faeces. Really, not very much power at all. [1] Following on from this, [15] describes British prototypes demonstrated at Glastonbury, where the power produced in the toilets powered the lights and charged phones. Unlike most of the other links on this page, this piece is readable, so do.
One version of MFCs is for desalination. Most of the current routes to desalination consume vast amounts of energy, so any route that reduces this is considered a big step forwards. The trick here is that the bacteria at the anode produce protons much as before, the membrane doesn't pass protons, so that negative ions move to the anode chamber. As the cathode takes the protons so positively charged ions move to the cathode chamber. So a three chamber vessel will desalinate the salty water in the middle chamber. [10] [11] This works very well as a way of reducing nitrogen in run-off from fields, [11] and gives us the term SMDDC to describe the submerged tank that does both denitrifaction and desalination. I susp[ect it needs a small voltage across the terminals (under a volt) for optimum performance. What I found to read continued to imply that power was produced, but not what was achievable with that. Source. what seems most effective in desalination is to use MDCs as a precursor to electrodialysis, an early part of the process, becasue the membrane is soon fouled, which implies to me that one needs a set of precursor tanks, some of which are always being somehow cleaned for re-use. [11] says 5000 hours (six months, roughly) is a reasonable time before the fouling is sufficiently bad to spoil the effect. The same source indicates this reduces energy consumption by approoaching 50% and saves a quarter of the time. Again, the implication that energy is produced is implied but not detailed, so one assumes it is low. I didn't discover what happens to salt (brine) produced and I expect there are small amounts of gaseous hydrogen and chlorine to deal with. There are some interesting comparisons to work through to choose whether ion exchange will be more cost-effective than desalination, but I didn't find the level of detail that would allow the creation of some practical problems. [12]
There are many ways of using MFCs to abstract heavy metals from contaminated material, but imagine this mostly being organic or in solution. In some of these there is elctron transport at both anode and cathode, specifically transport can occur at the anode where this electrode accepts electrons from microorganisms, but it can also occur at the cathode where microorganisms accept electrons from this electrode. [13] The diagram to the right here assumes that no external power consumption occurs; the process is spontaneous. Again, I found no indication whether there was power in excess. I think I'm missing some physics/chemistry here; back when in teaching, I'd go find a subject specialist and ask; now I can't do that. ²
I found myself wondering if a biobattery would be appropriate in medical uses, for example to power some metering device situated inside a body cavity, so that a flow of blood, for example, provided enough sugars to power some device with very low demands, but to keep it going for a very long time. I read of the electrodes being very thin plates (think nanometres thick, 3D printed) and that led me to such pondering. I'll hope one of my past pupils can respond.
_____________________
Battery warehouses are proposed and occurring, where capacity is measured in gigawatt hours. The 1.3 GW-h proposed at [8] made me wonder what the anticipated life of such buildings is thought to be. How much are we prepared to pay for storage, how do you cost it, so when is the building as a unit cost-effective or making a return on investment? What is the life of one cell among so many? I have no idea how one might calculate that in advance of initiating the building. I can imagine the desperate need for such modelling to be accurate.
Among the ideas is this: There is also developing vehicle-to-grid technology that aggregates many electric vehicles and enables bidirectional charging to essentially use them collectively as one huge battery. Currently a trial called Bus2Grid is underway in London. As the batteries are owned by whoever owns the vehicle, and so are “free” to the grid, this potentially offers the lowest cost storage. However it is yet to develop at a larger scale and will require lots more people to switch to electric vehicles.
I also wondered how much the price of lithium has to move before the alternatives become more attractive.
__________________
In the same way that this page describes a load of functions where microbiotcs are generating small amounts of power, one has to wonder whether add a small voltage across a biologic porcess can accelerate that process. This might describe what is occurring in the desalination above and quite clearly applies to anaerobic digestion, where a the yield from a process called electromethanogenesis (linked video) can be quite significantly improved by putting a voltage across the unit (which then of course is deemed to be a cell as in battery cell). Look up (mic)Robial and Plant-e, who provided the inset flower power picture that lights a small bulb. Room for many puns here. It is not clear how much work is involved in the manufacture or installation of the electrodes so, while they refer to using a rice field to power something significant, taht hasn't explained how much work is required beforehand, nor the maintenance of the components. Watch this space, perhaps. A 100x50 cm crate of plants lights a very few LED lights. I see that the laying of anode requires digging to depth of around a half metre and I'm entirely unsure how the cathode works. This clearly will work better in wet land.
_______________________
I had a brief look at other sorts of battery and these may turn into further pages. Of interest but as yet with not enough content to make much sense of, are:
The Quant E limosine, nanoflowcell drive. Th ebattery has two separated salt-water supplies, charged witj opposite polarity and the combination prooduces prodigious power. There advantages are that refilling is as quick as fossil fuels and zero tixicity, but no-one is explaining how the fluids are charged or how that is costed.
Tesla is talking about a jump in battery range between recharges that will exceed a conventional car by multiples; the Tesla 3 achieves 300 miles between charges but the new version talks about taking this past 1000 miles. The 1865(0) Ni/Co/Al (NCA) cell found the Tesla S gives 200 miles. The later 90kWh battery with improved cell chemistry added silicon to the anode. The model 3 uses 2170 cells (that's diameter and height in cm), this adds 50% energy and 100% power. The energy density of the two cells is similar, 240-250Wh/kg. This results in a dramatic reduction in 'pack' weight (the volume of battery used in a car model) of 21%, down to 470kg and around 160Wh/kg. Then there is the 4680, a far larger cell size, which gives 5x the energy, 6x the power and so +16% in range. Economies of scale make the larger battery quite a bit cheaper to make, perhaps down by 50%. So the jump in energy at the level of the individual cell is to 80-90Wh per cell. This will translate to a jump of about 15% improvement for the same size battery pack. This (Tesla battery day report) suggests a range improvement towards 500 miles between charges and the packs may retail in 2023. Tesla is talking about a battery that lasts a million miles, recharges in 1o minutes and exceeds 1000 miles between recharges; nice round numbers for targets. Moving away from cobalt and lithium is an attractive proposition.
Lithium-air batteries: Specific energy high, recycling is as yet a problem.
Nanotechnology, Redesign of the structure at a very small scale looks like it will allow the identified problems of batteries, such as crystal growth (dendrites), to be accommodated. This may include using carbon nanostructures as a sort of base plate. Watch the company called Amprius, who seem to be on a winner.
Lithium Sulphur, In theory, 2567 Wh/kg compared to Lithium Ion 350 Wh/kg a 7-fold improvement if we achieved the same efficiency. Search on Alise. This puts sulphur as replacement for the cobalt and manganese we have been using (and the political ramifications of the mining of those metals).
Solid State - swap flammable organic liquids for glassy-state solids or polymer base. Search QuantumScape.
Dual Carbon - both electrodes of carbon and a non-toxic electrolyte sound wonderful. Search PJPEye. At the moment likely to be bigger and heavier than the Li-ion equivalents for the same performance. However, the battery behaviour is different and that makes the technology attractive, offering a 40% increase in range over the comparable Li-ion battery. I cannot tell if that is a meaningful statement. Watch this space.
The need for better batteries is, mostly obvious. I'm keen to have a home-based battery that the solar panels output to, but as yet the price is ridiculous and uneconomic. The ability to recharge at speed (like 10 minutes) and with great repeatability (large battery cycles, like 5000 or more in the life of the battery) are things we wouldn't have declared as wants 50 years ago, but now we're looking for nation-sized batteries and in huge volume, such that the material we use are becoming part of the issue.
I will keep watching and hope to expand / extend reporting on the topic.
DJS 20201222
Some additions made, 20210127.
Idea new to me in Science 20191204 - to follow up. Added as [17] Blue energy, iontronics. It struck me that this might work very well where sea ice meets water; I'm bothered about the flow in rivers, so the idea of putting these where fresh water meets salt is fine, but not at every river mouth, though maybe it would work on a large delta.
Arguments for and against hydrogen (vs batteries) are made here. Hydrogen does not win for several reasons; it isn't as green as you might expect, it is surprisingly (energy) inefficient across the whole process and it is quite a bit more expensive. Yet Japan has committed massively to its development. The video is 15 minutes long.
top pic: Graphene the next big thin(g)?
1. Glucuronolactone is a popular component of energy drinks, it is created from glucose in the liver (suggesting a possible short story in which internal electrical gadgets such as memory storage are run off one's liver, Liver goes Live!). It is an important structural component of nearly all connective tissues (wikipedia).
2 I have been finding units of mW/m² to describe power density. I don't understand this, because it strikes me that it ought to be basically Watts per volume, not Watts per area.or watts per mass unit. Milliwatts, mW, are small. perhaps what is meant is surface power density, but that also doesn't seem to apply well to battery technology, unless it refers to the power produced at anode or cathode. When I tried to look this up, it seemed confined to beams, meaning laser light, where W/cm² would be a sensible unit. Searching within battery technology I found this image of energy density.
[2] https://www.sciencedirect.com/topics/biochemistry-genetics-and-molecular-biology/microbial-fuel-cell
In MFCs, the electrons released by bacteria from the substrate oxidation in the anode compartment (the negative terminal) are transferred to the cathode compartment (the positive terminal) through a conductive material. In the cathode, the electrons are combined with oxygen and the protons diffused through a proton exchange membrane. MFCs require sustained electron release in the anode and electron consumption in the cathode.17 The attainable metabolic energy gain for bacteria is directly related to the difference between the anode potential and the substrate redox potential. The optimal design for MFC is still under investigation, and different materials for the electrodes as well as more selective membranes for proton exchange are being currently developed to enhance their performance. [2]
The feasibility of domestic wastewater treatment by MFCs has been successfully tested in laboratory experiments, obtaining COD removal up to 50% and power densities about 420–460 mW m−2.2 Recently, C and N removal was obtained in an MFC fed with synthetic wastewater containing [ammonium ions] and acetate. Here, SND was performed in the cathode compartment separately from carbon oxidation.27 This configuration optimised the C source and reduced the COD requirements. Moreover, as denitrification uses the electrons obtained from the separate oxidation of organic matter present in the wastewater, the MFC system can operate very efficiently at low COD/N ratios. This reduces the requirement for an external C-source supply. Thus, the results reported in the literature prove that N removal with electricity production in MFCs is possible and bring the horizon of self-sufficient WWTPs closer. [2]
[3] https://www.sciencedirect.com/book/9780128163283/recent-developments-in-applied-microbiology-and-biochemistry covered in [2], fortunately. MFC, as energy-saving technology, may well wean for us far from the dwindling oil assets. Before that, there are many technical challenges that must be considered for sustainable and renewable energy generation.
[4] https://www.sciencedirect.com/book/9780444521149/bioprocessing-for-value-added-products-from-renewable-resources As [3], covered in [2]
[5] Wikipedia entry. https://en.wikipedia.org/wiki/Microbial_fuel_cell
[6] https://vtechworks.lib.vt.edu/handle/10919/64969 suggests that the use of sediment MFCs is restricted to the decontamination of wastewater. What we want tot read is of wastewater generating surplus power and feeding this to the grid.
[7] https://en.wikipedia.org/wiki/Biobattery
[8] https://theconversation.com/huge-battery-warehouses-could-be-the-energy-stores-of-the-future-151653?utm_medium=email&utm_campaign=Latest%20from%20The%20Conversation%20for%20December%2023%202020%20-%201820917698&utm_content=Latest%20from%20The%20Conversation%20for%20December%2023%202020%20-%201820917698+CID_2e2dd127c5cf5236e702c1dfa9ff397e&utm_source=campaign_monitor_uk&utm_term=Huge%20battery%20warehouses%20could%20be%20the%20energy%20stores%20of%20the%20future
[9] https://www.frontiersin.org/articles/10.3389/fenrg.2019.00001/full
[11] wikipedia https://en.wikipedia.org/wiki/Microbial_desalination_cell
[12] https://web.archive.org/web/20070203184420/http://cape.uwaterloo.ca/che100projects/sea/ed.html
[13] https://www.frontiersin.org/articles/10.3389/fenrg.2019.00001/full
[14] https://www.frontiersin.org/articles/10.3389/fchem.2018.00050/full I failed to understand this at all. Challenge.
[15] Pee power !! https://power.nridigital.com/power_technology_mar19/a_new_source_of_clean_energy_urine_luck Readable.
[16] https://www.youtube.com/watch?v=ZdieLPQUpcw&list=RDCMUCRuCgmzhczsm89jzPtN2Wuw&index=1 video!!
The piece [8] in The Conversation had a lengthy response from Lewis Cleverdon, quoted in full below, that I'm having trouble demystifying.
In identifying the merits of a particular energy storage system it would surely be helpful to identify the full range of storage services foreseeably needed, so that the scope of the option in question can then be seen in context. In this light it is worth outlining a phenomena that will probably impose the top end of the energy storage requirement.
Unfortunately there is an aspect of Climate Destabilisation that is exacerbating the risk of loss of generation due to adverse weather (cold windless heavy cloud) for extended periods across very large areas (potentially at worst over a fortnight and Europe-wide). This arises from the increasing disruption of the Jet Stream which in turn results in “blocking highs” causing a particular weather system to reside over an area for an extended period. While such events would be of potentially massive impact in terms the need for back-up power (assuming expensive non-intermittent renewable sources will be few), those events’ occurrence may be less than once per decade.
The limitation on Li Ion Battery banks’ viable capex {??} per GWH of usable energy storage capacity (not nameplate) can be seen in the number of times per year some generation shortfall causes a system’s profitable net full discharge - For instance, a system discharging once per five days will earn massive revenue compared with a storage system that discharges only once per five years, and for the latter the battery systems discussed in the article would be viable structurally but plainly not financially.
From this perspective a ‘strategic energy reserve’ is required that comprises :
- a stock of a durable low-cost fuel and high-efficiency conversion systems,
- that are of a scale to replace Europe’s weather-dependent generators for a fortnight or more,
- and that is generating power often enough (serving grid demand) to cover its costs and turn over its fuel stocks appropriately.
This outline implies that there may perhaps be competition with Battery storage systems for market-share at the upper end of the latters’ viable capacity range, with the strategic energy reserve having the political advantage of being an imperative priority for which there is no alternative.
In terms of the fuel and technology for such a reserve, the reason the research quoted in the article found Li Ion car batteries to be the cheapest scaleable energy storage was that it excluded a traditional fuel-production technology that offers a highly relevant co-product and co-benefits: - the sylviculture of Native Coppice Forestry has provided feedstock for charcoal since prehistory, with the exothermic retorts’ hot hydrocarbon offgasses having been used for methanol [CH3OH] at least since the C17.
While charcoal now has a key role as ‘Biochar’ soil amendment both for globally raised crop yields and for Carbon Recovery, Methanol is an exceptionally stable liquid fuel that can serve 50% efficient HT PEM fuel cells. (Notably it avoids all the critical demerits of liquid hydrogen of high production & compression energy costs, of the embrittlement of steel, of very high tankage weight, volume & cost, daily bleed-off, extreme explosion risk, etc).
As such methanol offers valuable flexibility in meeting the needs of fuel cell transport for surface freight on road, rail and shipping, thus potentially easing the requisite turnover-period of methanol stocks held in strategic energy reserves. This again indicates that while Li Ion Batteries will doubtless play an outstanding role in grid energy storage, there are a range of storage roles for which they will not be appropriate.
Update Sep 2020. https://www.youtube.com/watch?v=DOBBwx3Cbbk offers so me good comparisons; Li-ion batteries 0.3 MJ/kg vs. gasoline 48J/kg. Li-S batteries 'offer' 2567Wh/kg vs. LI-ion 350Wh/kg maximum. [1Wh=3.6MJ, 1 MJ= 277.8Wh, but Wh are a better measure for many of these uses, since the speed of delivery of energy is the issue, not how much there is.] So a switch to Li-S might save 7 times the weight or give seven times the range. Sulphur would replace the Co and Mn, so there's a serius ,oney saving too. ALISE has a target of 500Wh/kg. There are issues to resolve.
Tesla 3 now at 380 miles between recharges. Toyota think they have a solid-state battery for 2021, taking range to 500 miles and adding 300 miles in ten minutes. Please check this. As batteries drop in price (inset), so do the cars and 2021 may see a mass market change. I suggest that the measure of charging will be (is already) miles of charge in minutes at the charger. I think we might have a standard unit of a hundred miles in one minute, a cento-mom perhaps. At that speed, there's as much time lost in faffing around getting the plug into the car as there is wait while it does its thing. Search on StoreDot.
Look at price parity (between ICE and EV) here, predicted to occur in the UK 2023-25. I noticed an issue about EU/UK that requires UK sources of battery materials; it may only be a potential issue since the whole article could be written off as froth.